Dice, Walls and Boosters
Two opposing interpretations of the evidence about waning vaccine protection are grounded in two very different models of the course of a Covid-19 infection. In two masterful tweets, John Burn-Murdoch captured and named them: the dice model and the two-walls model.
Under the dice model, waning protection against infection implies waning protection against severe disease. Because there are fewer instances of more serious outcomes, it is more difficult to tease out statistically significant evidence of waning against a more serious outcome than against infection. But if the dice model is right, the two always go together.
Under the two-walls model, it would be possible to have waning protection for infections without waning protection for severe disease, but there is a problem with this model. Part of the beauty of the stripped-down characterization by Burn-Murdoch is that it is precise enough to surface its intrinsic logical contradiction. There is a way to patch the model to remove this contradiction, but what remains is a biologically implausible model that is starkly inconsistent with the data.
As a result, the the default presumption should be that statistically significant evidence of waning against infection also implies waning against more serious outcomes. This presumption could be overturned by tight estimates that show no waning of protection against these more serious outcomes, but not by low-powered tests that generate big uncertainty intervals.
1. The Tweet Versions of the Two Models
Because Burn-Mudoch’s charicatures are so pithy and memorable, they are worth quoting verbatim: Model 1?

If this Dice model is true, the laws of probability imply that the expected number of more serious outcomes increases when the when waning protection increases the number of people who pass the first stage in the process, infection. Hence, it is impossible to have waning protection against infection and without waning protection against severe disease. Because the number of cases of severe disease is lower, it will be hard to get statistically significant direct evidence of waning protection against severe disease, but the logic of the model means that when the number of infections is increasing, the expected number of cases of severe disease is also increasing.

Under the model with two-walls, the virus may breach the first wall and cause more infections with no effect on the number of people who get severe disease because there is a second wall that prevents any of the additional infections from becoming severe.
2. Formalizing the Two Models
In a large group with given characteristics, let ||I|| represent the number of people who get infected and let ||S|| be the number who get severe disease. Before we know what happens, we can put a tilde over each letter to show that ||\tilde{I}|| and ||\tilde{S}|| are random variables characterized by a joint probability distribution. Once we know the number of infections, ||I|| is a number. Given that value for ||I||, we have a conditional distribution of the random variable ||\tilde{S}|| for the given value ||I||.
One can then calculate a function that yields the expected value of ||\tilde{S}|| for each possible value of ||I||. To represent this, let an overbar denote the expected value. Then ||\bar{S}(\cdot)|| is a function and ||\bar{S}(I)|| is its value for a given number ||I||.
The crucial difference between the two models is that under the dice model, ||\bar{S}(I)|| is an increasing function of ||I|| but under the two-walls model, ||\bar{S}|| is a constant that does not depend on ||I||.
More formally, the two-walls model assumes that ||\tilde{I}|| and ||\tilde{S}|| are independent random variables. The realized value of ||\tilde{I}|| is determined by the first wall; the realized value of ||\tilde{S}|| is determined independently by the second wall.
The dice model recognizes that there is a sequence to these events. It starts with a random process that determines the number of people ||I|| who get infected. For each of these people, there is some probability ||p|| that their infection will develop into serious disease. The value of ||p|| will vary across members of the group. Conditional on being infected, someone who is older or who has other health problems will have a higher probability of developing severe disease. But as long as ||p|| is greater than zero for each individual – that is, as long as there any chance that each infection can develop into severe disease – each additional person who is infected increases the expected number of cases of severe disease.
3. Why the Difference Between the Two Models Matters
From a number of different studies, a large body of evidence has emerged that the protection the Pfizer vaccine offers against infection is falling. Under similar conditions, a larger fraction of the more people who are double-vaccinated with Pfizer are getting infected now.
It is harder to measure what is happening to the protection that the Pfizer vaccine offers against severe disease, so policy discussions are forced to rely on a model to answer an “if .. then” question: “If ||I|| increases, what happens to ||\bar{S}||?”
If the dice model is right, more infections implies more cases of severe disease. This means that if protection against infection is falling, protection against severe disease must also be falling.
In contrast, if the two-walls model were correct, protection against infection could fall and cause more infections, with no change in the number of cases of severe disease. As a result, we could see falling protection against infection with no change in protection against severe disease.
4. The Two-Walls Model Is Logically Incoherent
There is a simple fact which tells us that the two-walls model cannot be right: It is impossible to get a severe Covid-19 infection without having a Covid-19 infection,
This means that in any group, $$S < I$$ As a result, the value of ||I|| tells us something about the possible values for ||S||. If ||I|| is a very small number, then ||S|| must be as small or smaller. This means that the assumption that ||S|| and ||I|| are independent random variables contradicts the very definitions of these variables.
If there are any circumstances in which ||\bar{S} > 0||, it follows that when ||I|| is very small, the expected value ||\bar{S}|| must increase as ||I|| increases. For example, when ||I = 0||, ||\bar{S} = 0||. If ||I = 1||, it is possible that ||S|| could turn out to be ||1|| so ||\bar{S} > 0||. The same logic applies until we reach a value of ||I|| with the property that for all subsequent infections, there is zero chance that any of them will turn into a severe infection.
5. The Modified Version of the Two-Walls Model is Inconsistent with the Data
There is a modified version of the Walls model that can still yield the prediction that in some circumstances, more infections does not mean more cases of severe disease.
Suppose that we expect that a plot of ||\bar{S}(I)|| as a function of ||I|| will look like this:
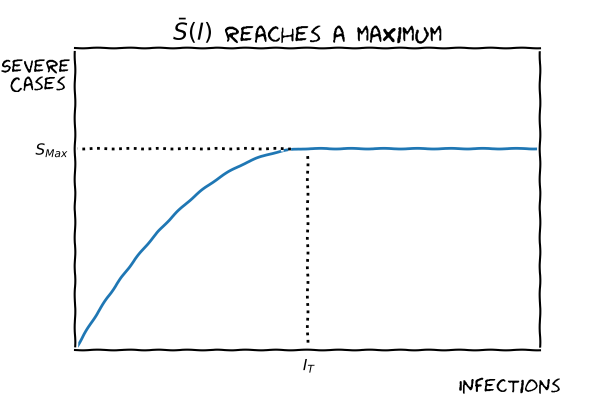
For low enough values of ||I||, an increase in ||I|| does imply an increase in ||\bar{S}(I)||, as it must if there are ever any cases of severe disease. But there could be a threshold value ||I_T|| where the number of cases of severe disease reaches its maximum value ||S_{Max}||. For larger numbers of infection, ||\bar{S}(I)|| remains constant.
If there were a maximum value for the number of cases of severe disease, and if the number of people who are infected means that ||S|| has already hit its maximum, then as protection against infection wanes and even more people get infected, it is possible that the number of cases of severe disease will not change. Under these circumstances, it is possible that protection against infection falls without any reduction in the protection against severe disease.
This extension implies a biologically implausible form of dependence between the outcomes for different people. If more infected people have already developed severe disease, this reduces the probability that other infected people will develop severe disease.
But the more serious problem with this version of the two-walls model is that is no evidence that supports a maximum value ||S_{Max}||. In every case where we have seen a big change in the number of infections, we have also seen a change in the same direction in the number of hospitalizations, cases of severe disease, and deaths.
6. The Puzzle
Given its logical and empirical inadequacies, it is hard to understand why a regulatory decision about who is legally allowed to receive a third dose of a vaccine could hinge on the argument that the two-walls model is, nevertheless, the right model to use to interpret the available data on the changing protection offered by vaccines.